Once upon a time, circa 2700 BC in China, empress Xi Ling Shi was enjoying her afternoon tea under a mulberry tree, when a silkworm cocoon fell from the tree into her tea. She noticed that on contact with the hot beverage, the cocoon unraveled into a long silky thread. This happy accident inspired her to acquire these threads in abundance and fashion them into an elegant fabric.
So goes the legend, according to the writings of Confucius, about the discovery of silk and the development of sericulture in ancient China. Although archaeological evidence from Chinese ruins dates the presence of silk to 8500 years ago, hinting that the royal discovery story was spun just like the silk fabric, one part of the legend rings true.1 The Chinese royals played a pivotal role in popularizing silk as a symbol of status and wealth. By 130 BC, emperors in the Ancient Civilizations across the world desired to be clad in silken garments, paving the Silk Road that opened trade routes from China to the West.
While silk maintained its high-society status over the next thousands of years, the demand for easy-to-use materials grew among mass consumers. In the early 20th century, textile developers applied their new-found technological prowess to make synthetic materials: petrochemical-based polymer blended textiles with improved durability, strength, and convenience.
Today, the textile industry is witnessing a new kind of synthetic revolution, one that paradoxically advocates bringing back natural materials. Synthetic biologists are using natural systems to produce materials with enhanced features to usher in an era of sustainable fashion.
Spider Spidroin Revives the Silken Splendor
In their quest to make silk powerful again, not by status but rather by thread strength, scientists turned to an arachnoid. Dragline silk, the thread by which the spider hangs itself from the web, is one of the strongest fibers; its tensile strength—a measure of how much a polymer deforms when strained—is almost thrice that of silkworm silk.2
Beyond durable fashion garments, tough silk fibers are coveted in parachutes, military protective gear, and automobile safety belts, among other applications, so scientists are keen to pull on these threads. While traditional silk production relies on sericulture, arachnophobes can relax: spider farms are not a thing.
“Spiders make very little silk and are quite territorial. So, the only way to do it is to make microbes that make the protein,” said David Breslauer, cofounder and chief technology officer at Bolt Threads, a bio apparel company.
David Breslauer is the cofounder and chief technology officer at Bolt Threads.
bolt threads
For decades, researchers have coaxed microbes into churning their metabolites in large fermentation tanks, which they have harvested to solve dire crises in many areas. For instance, when pharmaceuticals struggled to meet the growing demand for insulin through the traditional methods of extraction from animal pancreas, researchers at Genentech sought the aid of E. coli to generate recombinant insulin for mass production in 1978.3
However, researchers soon realized that producing spider silk in microbes was no easy feat. The spider silk protein, spidroin, is larger than 300 kDa in size—a huge jump from the small 6 kDa recombinant insulin. Bulky proteins impose a heavy metabolic load on the microbes and their production yield tanks. Also, spidroin consists of repeating regions of glycine and alanine amino acids that impart strength and elasticity to the material, but the host microbes struggle with protein folding and overexpression of the corresponding tRNA molecules.4
Around 2013, Fuzhong Zhang, a synthetic biologist at Washington University in St. Louis, came across an article about the challenges of spider silk protein synthesis. Zhang, who had freshly started his own lab after working on metabolic engineering during his postdoctoral research with synthetic biology pioneer Jay Keasling at the University of California, Berkeley, saw potential in the project. “I was excited about the material’s properties and also excited about the opportunity that provided to synthetic biologists,” said Zhang.
At the time, researchers had gotten close, but they hadn’t been able to synthesize the full spidroin protein. Since the molecular weight of the silk protein correlates with the strength of the silk thread, Zhang was determined to produce the entire protein to mimic the silk’s natural properties.5
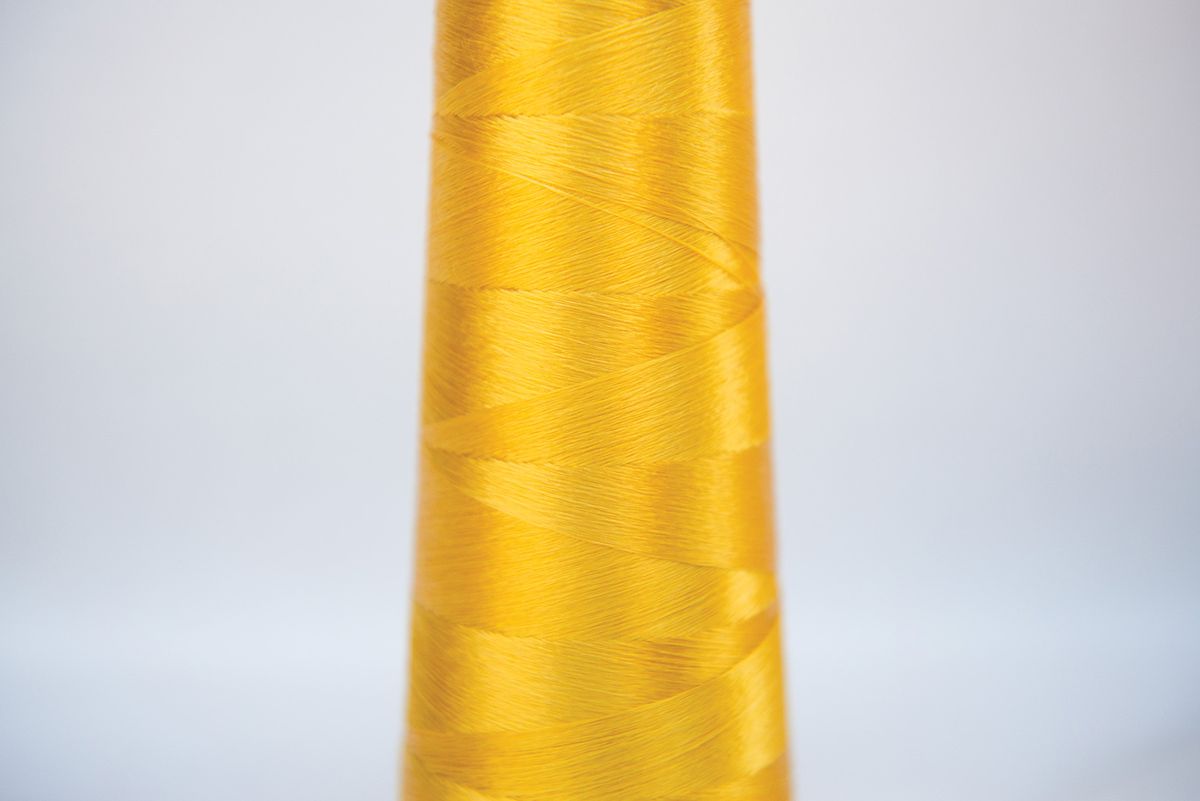
The team at Bolt Threads developed Microsilk, a spider silk inspired biomaterial.
bolt threads
To achieve this goal without pushing the metabolic limits of the bacteria, Zhang and his team literally broke down the problem. In 2018, they devised a recombinant spidroin by constructing two protein halves with split inteins—peptides known to catalyze ligation between proteins while splicing out their own residues—tagged at their ends. They synthesized the halves in separate E. coli cultures, mixed the two cultures, and ligated the proteins to yielded a recombinant spidroin of 556 kDa—a size that was previously considered unobtainable.6 The resulting silk fiber made from these recombinant spidroins matched the mechanical properties of natural spider silk fiber.
“That was the first report demonstrating that you can make such a fiber from an alternative host,” said Zhang.
While synthesizing the high molecular weight protein validated their technical prowess and strategy, Zhang knew that the yield with this approach was going to be unavoidably low. “It was not even enough to make a simple shirt,” he said.
Zhang and his team started looking into strategies that would allow low molecular weight proteins to work in a way similar to high molecular weight ones to increase their yield. On exploring the structure-property relationships within the spidroin sequence, the team focused on two parts of the protein. The sticky terminal domains, which join end to end create long polymers, and the repeating peptides in between that fold into beta sheets that are crucial for fiber strength. They tinkered with sequences from different proteins to generate a spidroin functional mimic.
Finally, something stuck. In 2023, they engineered a mussel foot protein (secreted by mussel for underwater surface adhesion) at the terminal ends of spider silk repeats, producing a material that was lightweight but stronger than the natural spider silk threads.7
There was so little innovation in the textile space, and brands were really eager to talk about innovation. It felt like there was demand there. Turns out, the desire for storytelling outweighed the desire for actual innovation with those brands.
—David Breslauer, Bolt Threads
Next, Zhang wants to translate these technologies into industry. “That will involve scalable and cost-effective production of these materials for real applications,” said Zhang.
Breslauer echoed the importance of this step. He recalled how scaling up was the biggest challenge when he and his cofounder Dan Widmaier, chief executive officer at Bolt Threads, first set up shop in 2009. The duo met during their graduate studies. Breslauer, a material science student at the University of California, Berkeley, was fascinated by spider silk and sought help for synthesizing the protein in microbes. Luckily, he met Widmaier, a synthetic biology graduate student who was optimizing systems to study complex proteins.
When their collaboration to produce recombinant spider silk proteins in yeast yielded promising results, the duo decided to challenge the status quo in the textile industry by commercially producing bio-silk apparel, and Bolt Threads was born. The market transition, however, was not as smooth as the threads they produced.
“There was so little innovation in the textile space, and brands were really eager to talk about innovation. It felt like there was demand there. Turns out, the desire for storytelling outweighed the desire for actual innovation with those brands,” Breslauer said. “We didn’t realize how adverse people were going to be to the idea because it was so unfamiliar.”
Yet, they persisted, and over the years collaborated with established brands and designers on garments made from recombinant spider silk and other biomaterials.
See also: “Infographic: Synthetic Biology is Transforming Clothes and Cosmetics“
Leather That Need Not Hide
Unlike its classy silk brethren, leather had a rough start. When primitive humans roamed tough terrains, some innovator probably pitched the idea of draping animal hide on their skin as a protective layer. Sometime over the next thousands of years, leather’s repute transformed from a pragmatic covering to a highly coveted material, despite its hideous production process.
David Williamson, a chemist and the chief operations officer at Modern Meadow and his team wanted to separate themselves from the herd. In their quest for sustainable alternatives, they went back to the basic biology and chemistry of the material. As leather is made from animal skin, it is rich in collagen, a structural protein abundant in the extracellular matrix of connective tissues. If the team could produce this primary component protein at scale, they would be able to process it into leather downstream.
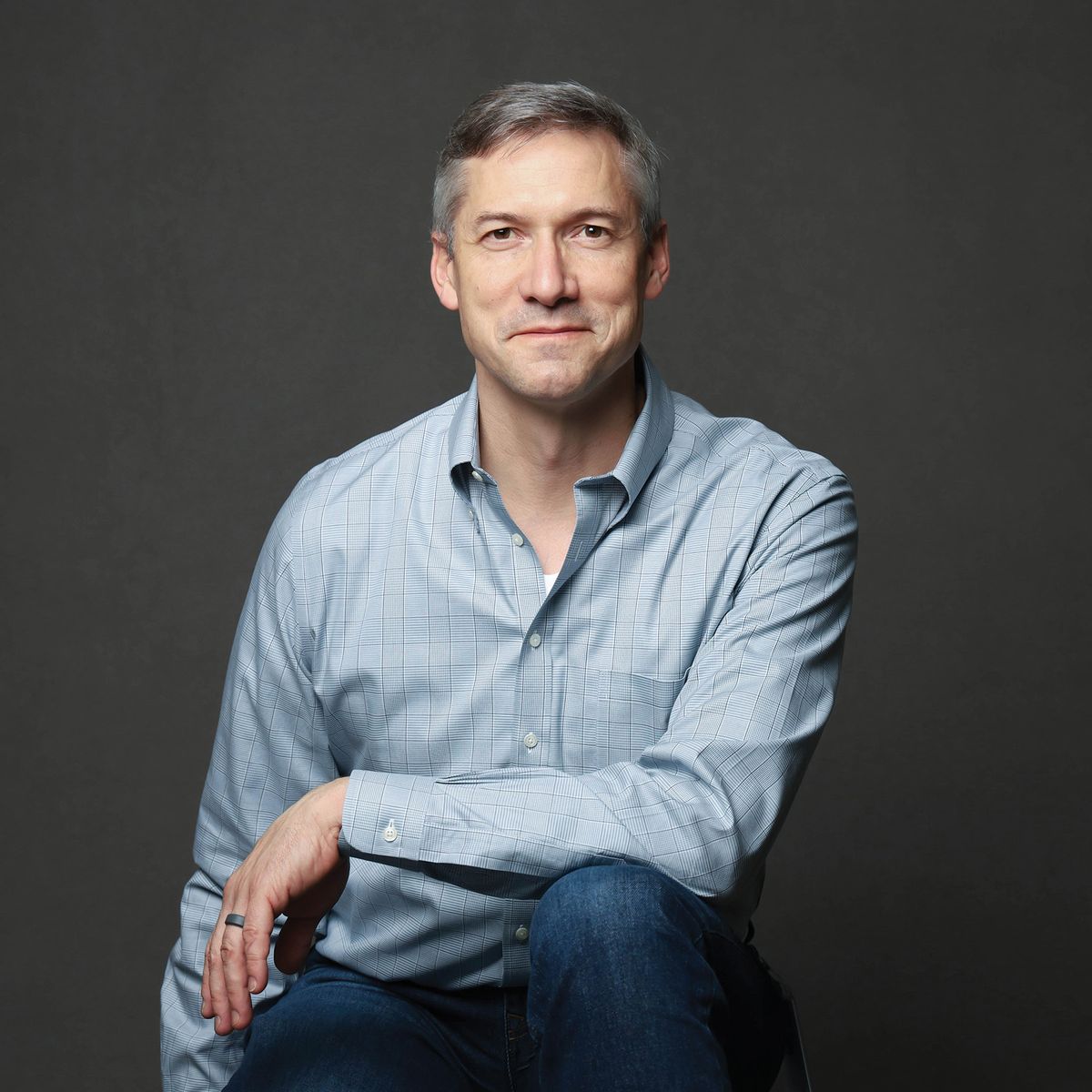
David Williamson is the president and chief operating officer at Modern Meadow.
modern meadow
In about 2017, Williamson and his team developed a fermentation-based approach to produce collagen from yeast. While they achieved scalable production, there was one small hiccup. The protein properties of collagen alone did not yield the mechanical properties they needed for their leather-like material.
“We started to think about, ‘okay, how can we augment what the protein delivers with something that’s going to work from a polymer perspective, so we bring the best of material science with the best of synthetic biology?’” recalled Williamson.
The team went to the drawing board and analyzed the amino acid residues that contributed to collagen’s characteristics to look for a substitute protein. They found an alternative that had the desirable functional elements of collagen but was also sustainable and cost effective for industrial scale up: soy protein isolate. While tinkering with their recipes, they found the perfect combination for material strength by mixing in a bio-based polyurethane polymer with the protein to yield a refined bioalloy called Bio-VERA.
According to Williamson, Bio-VERA structurally resembles animal-derived leather, but it excels in performance. “Kangaroo is considered to be the toughest, strongest leather because of the way the fibers are laid down,” he said. So, the team conducted a flurry of tests to compare their material to the gold standard.
“When you run or you walk, the front end of your shoe will bend, and it will create a crease across that. Bally Flex is a test that’s meant to kind of assess a material’s suitability for that kind of a deformation,” Williamson explained. Bio-VERA exceeded the kangaroo leather performance for this test.
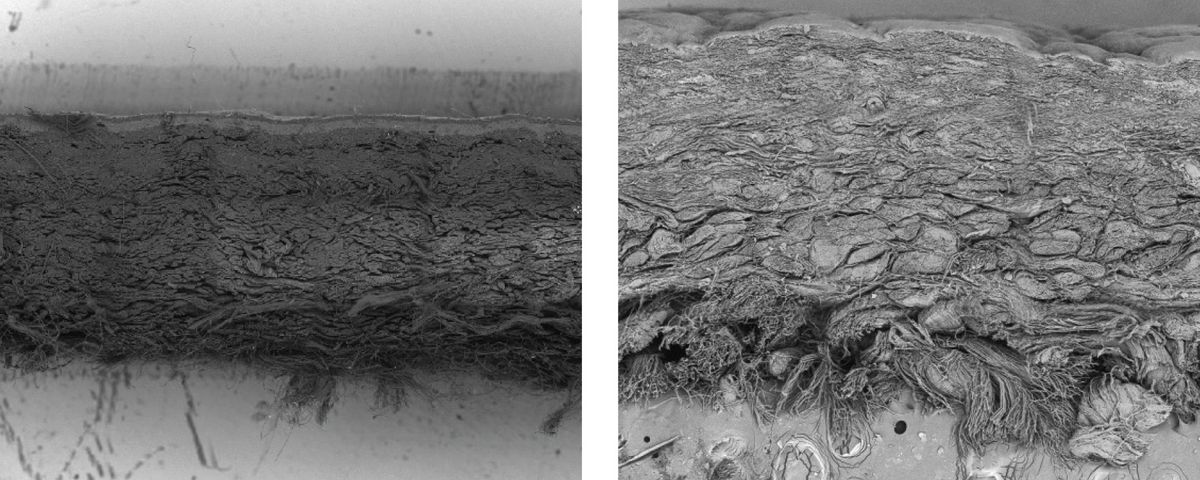
Modern Meadow researchers developed Bio-VERA (left), which is a bio-based fabric that is engineered to be similar to leather (right), as seen from the cross-sectional SEM images.
modern meadow
Williamson is excited about the applications of the strong fabric beyond the apparel industry; he noted that the team is actively working with automotive brands to advance this material into the market.
Strands That Tighten the Skin and Strengthen the Hair
As natural textiles are derived from animal skin, hair, or proteins, it is no surprise that many synthetic biologists in the textile space have also found a niche in cosmetics. Even as the Modern Meadow team transitioned away from their protein fermentation strategies to innovate Bio-VERA, they realized that they could still apply their expertise in skincare. While leathery is not an adjective one desires to associate with skin, collagen is an integral component in both. “When our bodies make collagen and build our extracellular matrices, one of the first proteins that they deposit is type three collagen. So, you can think of type three collagen almost like the structure or scaffold of a building,” explained Williamson.
We decided that it would be a really good idea to transfer some of the knowledge that we had in wool textiles to human hair.
—Artur Cavaco-Paulo, the University of Minho
As cells layer type one collagen, elastin, and fibronectin on top, type three collagen guides their organization. As humans age, the amount of type three collagen in their skin reduces, altering the ratio of type one and three collagens. As a result, the skin loses its elasticity.8
To cater to the increasing demand for solutions to achieve younger looking skin, Williamson and his team engineered a recombinant collagen type three protein containing part of the protein sequence that is rich in binding domains for fibroblast interactions.9,10 “After you expose the extracellular matrix to this protein, it stimulates the fibroblasts to make more type three collagen. That type three collagen lays down type one collagen and elastin and fibronectin in a way that actually helps to turn back time, so to speak, to increase the ratio of type three collagen relative to type one collagen,” Williamson said.
The Modern Meadow team are not the only ones to weave their textile strands into cosmetic applications. When Artur Cavaco-Paulo, a biological engineer at the University of Minho, was studying wool fibers, he was struck by their structural similarities to human hair. “We decided that it would be a really good idea to transfer some of the knowledge that we had in wool textiles to human hair,” said Cavaco-Paulo. Particularly, he was interested in investigating solutions to fix hair strands damaged by highly alkaline chemical products.
Human hair is 80 percent keratin, a tough intermediate filament protein, by mass.11 In cross sections of human hair strands, keratin proteins line up, embedded in a matrix of keratin associated proteins (KAP) in the inner cortex layer, which is nestled within the outer protective cuticle layer of the hair strand. As the disulphide bonds within and between keratin and KAP are crucial for the strength of the hair filament, Cavaco-Paulo came up with the idea to use keratin-based peptides to spur disulphide bonds in damaged hair to improve the physical strength of the hair strands. “We went to do a really crazy project; it was looking at the whole human genome,” he said.
In the early 2010s, he and his team scanned the human keratin and KAP proteome to find peptides that fit their specific criteria, such as a minimum length of 10 peptides and high cysteine content. They stuck about 1300 of these peptides on glass arrays and tested for their affinities to human hair keratin. They shortlisted 10 suitable candidates for potential human applications.12
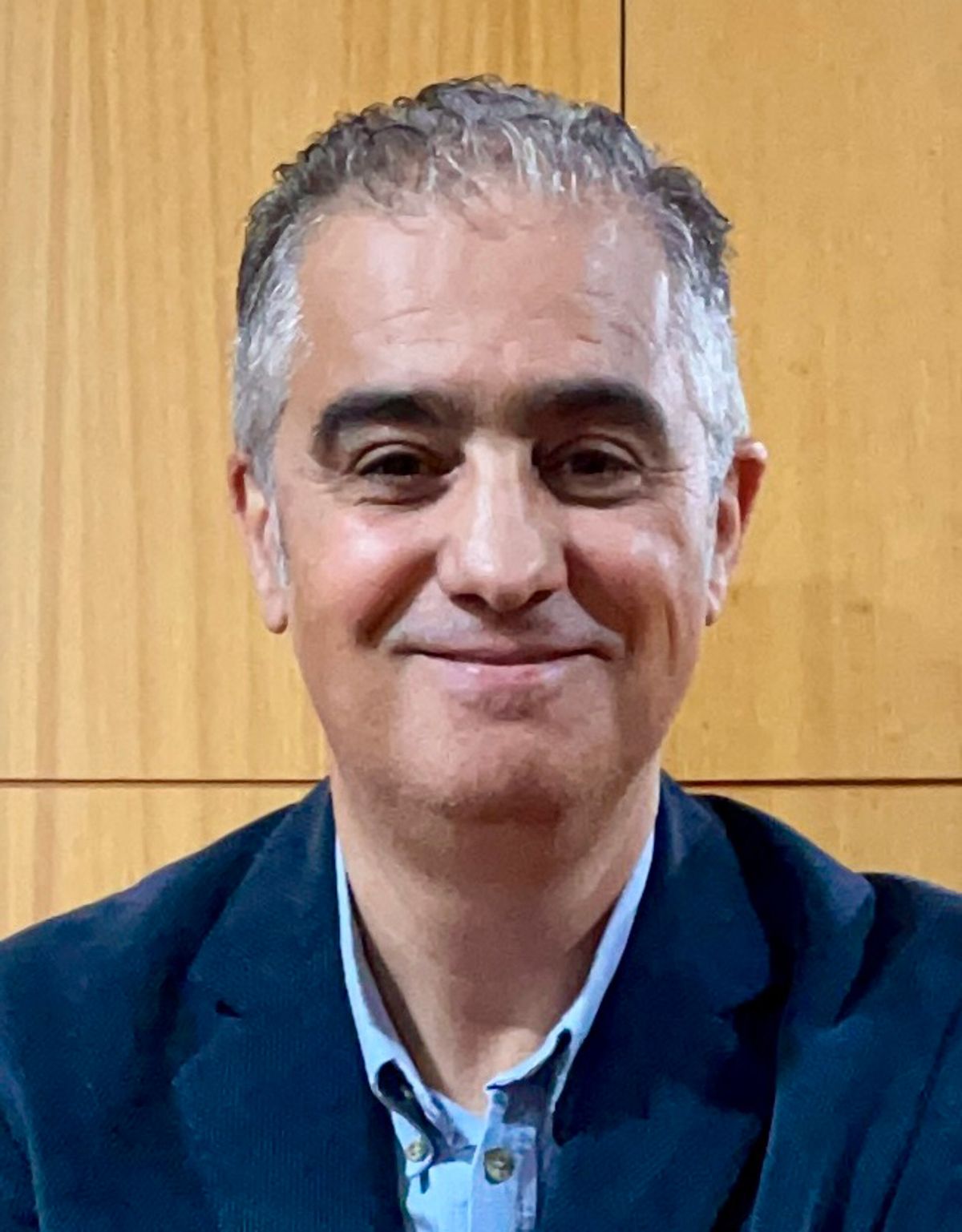
Artur Cavaco Paulo is a biological engineer at the University of Minho and the chief scientific officer at the biotech company K18.
Artur Cavaco Paulo
Over the next few years, Cavaco-Paulo developed one of these shortlisted peptides into the K18 peptide product, which is now part of a commercially available leave-in conditioner. Cavaco-Paulo serves as the chief scientific officer at the biotech company K18.
Although he started his career with textile research, Cavaco-Paulo favors the cosmetics sector with regards to returns on research and technology investment. “The personal care market is much more accustomed to innovation and has a much better and more fluid pipeline on innovation,” seconded Breslauer. “Whereas, [in] apparel, you really have to twist arms to get people to work with your material.” Bolt Threads ventured into the personal care space when Breslauer and his team serendipitously stumbled upon an alternative use for one of their textile proteins.
While he hopes to see more adoption of innovative biomaterials in the textile space in the near future, Breslauer believes that the complex downstream processing makes them a hard sell. For example, synthesizing the silk protein is just the first step towards making a fabric. Developers need to then spin it into a fiber, test the material properties, turn it into a textile, and then sell the end product. Every step associates with its own development, cost, complexity, risk, and quality control. “You’re building the plane while flying it. You’re still trying to build up the first step, and you’ve already promised that you’re going to sell the last step,” said Breslauer.
References
- Gong Y, Li L, Gong D, et al. Biomolecular Evidence of Silk from 8,500 Years Ago. PLoS One. 2016;11(12):e0168042.
- Shao, Z., Vollrath, F. Surprising strength of silkworm silk. Nature. 2002;418:741.
- Quianzon, C.C., Cheikh, I. History of insulin. J Community Hosp Intern Med Perspect. 2012;2(2):18701.
- Chung, Hannah, et al. Recent advances in production of recombinant spider silk proteins. Curr Opin Biotechnol. 2012;23(6):957-964.
- Xia, Xiao-Xia, et al. Native-sized recombinant spider silk protein produced in metabolically engineered Escherichia coli results in a strong fiber. Proc Natl Acad Sci USA. 2010;107(32):14059-14063.
- Bowen, Christopher H., et al. Recombinant spidroins fully replicate primary mechanical properties of natural spider silk. Biomacromolecules. 2018;19(9):3853-3860.
- Li J, Jiang B, Chang X, et al. Bi-terminal fusion of intrinsically-disordered mussel foot protein fragments boosts mechanical strength for protein fibers. Nat Commun. 2023;14:2127.
- Gao J, et al. Age-related changes in the ratio of Type I/III collagen and fibril diameter in mouse skin. Regen Biomater. 2022;10:rbac110.
- Li W, et al. Distinctive roles of fibrillar collagen I and collagen III in mediating fibroblast-matrix interaction: A nanoscopic study. Biochem Biophys Res Commun. 2021;560:66-71.
- Gelse K, Pöschl E, Aigner T, et al. Collagens—structure, function, and biosynthesis. Adv Drug Deliv Rev. 2003;55(12):1531-1546.
- Rogers MA, et al. Human hair keratin-associated proteins (KAPs).Int Rev Cytol. 2006;251:209-263.
- Cruz CF, et al. Peptide—protein interactions within human hair keratins. Int J Biol Macromol. 2017;101:805-814.